Investing in Natural Resources In a Changing World (Part I of V)
Primary Energy comprises commercially-traded fuels, including modern renewables, used to generate electricity (Source: BP Statistical Review 2019).
Issues to be addressed in this paper
Natural resources are crucial to humans. Water is the most important of them all, as we wouldn’t survive for more than a few days without it, but other natural resources are important too, none more so than our energy resources. This paper will focus on primary energy and seek to explain why the composition of it (see Exhibit 1) is likely to change profoundly in the years to come.

Note: mtoe = million tonnes oil equivalent
Source: BP Statistical Review 2019
To continue reading...
Ever since the early days of the industrial revolution, fossil fuels have been a critical ingredient in our desire for, and ability to deliver, higher living standards almost everywhere. It all started with coal-fired steam engines in Britain in the second half of the 18th century and now, some 250 years later, coal still plays a critical role in many countries – these days mostly as fuel in power plants.
Asia consumes more coal than any other region in the world (Exhibit 2) with consumption growing the sharpest in China and India. Coal’s share of primary energy consumption dropped to 27.2% worldwide in 2018, the lowest in 15 years, yet global coal production still rose by 4.3% (source: BP).
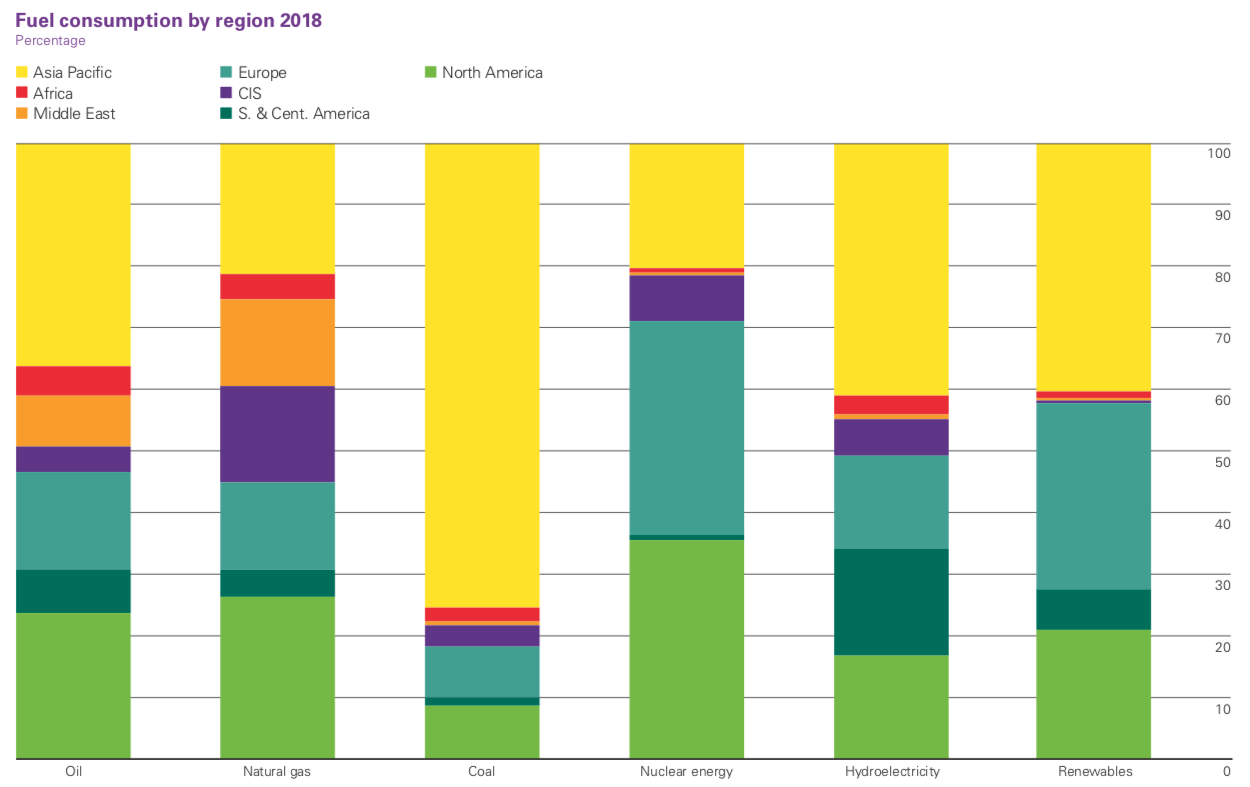
Source: BP Statistical Review 2019
The problem with fossil fuels – of which coal is only one – is that they do much damage to our environment when burned. According to the US Energy Information Administration (EIA), in 2017, carbon dioxide (CO2) emissions from burning fossil fuels for energy amounted to no less than 93% of total US anthropogenic CO2 emissions. With living standard rising rapidly across emerging markets – particularly in Asia – demand for energy will continue to grow. According to Scientific American magazine, global demand for energy will increase by no less than 50% over the next ten years and will nearly double by 2050.
Having said that, I believe fossil fuels are likely to be gradually phased out. As the Millennials age and gain in political influence, the attention to environmental issues such as the one pointed out by EIA above can only rise. I still come across those (mostly elderly) who argue that it cannot be proven that the ongoing climate change is man-made. The Danish queen is only the latest in a long string of people who are in denial. When I show them the chart below in response (Exhibit 3), at least some of them claim it is a hoax which demonstrates the challenge authorities are up against.
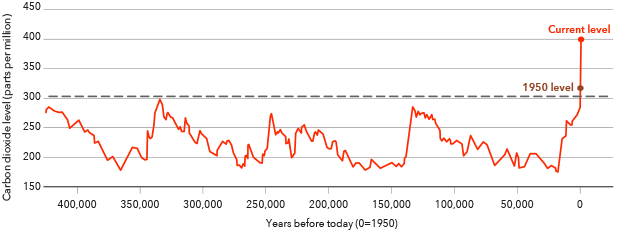
Source: BlackRock
One obvious implication of it all is that, across the world, authorities will increasingly restrict the use of the most damaging energy forms, and here fossil fuels stand out. In this research paper, I will try to identify which primary energy forms that are most likely to replace fossil fuels. In part II, I will look into which new, fossil fuel-free, energy forms there are in the pipeline (and there are indeed a few). Part III has been dedicated to water, as running out of freshwater is a rising problem around the world and, in part IV, I will look at other natural resources, that are likely to be in demand in the years to come. I will close this series of papers on natural resources with a paper on the opportunity set investment-wise.
Natural resources in the context of our megatrend approach
As you are probably aware, six megatrends drive everything we do at Absolute Return Partners, and two of those six megatrends will directly affect demand for natural resources for many years to come – Rise of the East and Climate Change. In the context of primary energy, rapidly rising living standards across Asia can only increase overall demand for energy, whereas a changing climate will force authorities all over the world to make alterations as to how those resources are utilised. We have identified a number of investment themes associated with those two megatrends (Exhibit 4), and they will be discussed in more detail in part V of this paper.

Source: Absolute Return Partners LLP
The three decisive factors when choosing energy form
Although some energy resources are used for a multiple of purposes (e.g. oil and natural gas), I will focus on power plants’ use of energy in the following and how that is likely to change. This limitation in scope makes sense from more than one point of view. Firstly, electricity production is a regulated industry, making it relatively easy for policy makers to change the framework. Secondly, electrification of all heating and transportation is already on the political agenda in many countries, i.e. power stations will inevitably consume more and more of our energy resources.
Three factors dominate the debate when policy makers decide which form of energy to utilise in power stations:
- the cost of the electricity generated;
- the carbon footprint; and
- various safety issues.
The cost of electricity
The cost of electricity varies dramatically from country to country, although prices tend to be the highest in Europe. MacroStrategy Partnership have found a statistically significant link between residential electricity prices and the penetration of wind and solar energy (see Exhibit 5), which may lead one to conclude that it is still too expensive to generate electricity from renewables like wind and solar.
If you then look at Exhibit 6, that doesn’t appear to be the case, though, so why are electricity prices the highest in those countries where wind and solar make up the biggest share of the power mix? I will address that question later but, before doing so, allow me to finish my thoughts on the three factors listed above.
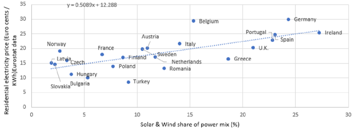
Source: MacroStrategy Partnership LLP
Note: based on data from Eurostat and BP
To begin with, let me introduce you to the concept of levelized cost of energy (LCoE). LCoE is a widely recognised measure of the cost of generating electricity. It measures the lifetime cost of generating electricity divided by total output, hence why it is measured in $/MWh. The most important drivers of LCoE are initial capital costs and the capacity factor (see definition later), but financing costs and annual operating expenses are meaningful drivers as well (Source: US Department of Energy).
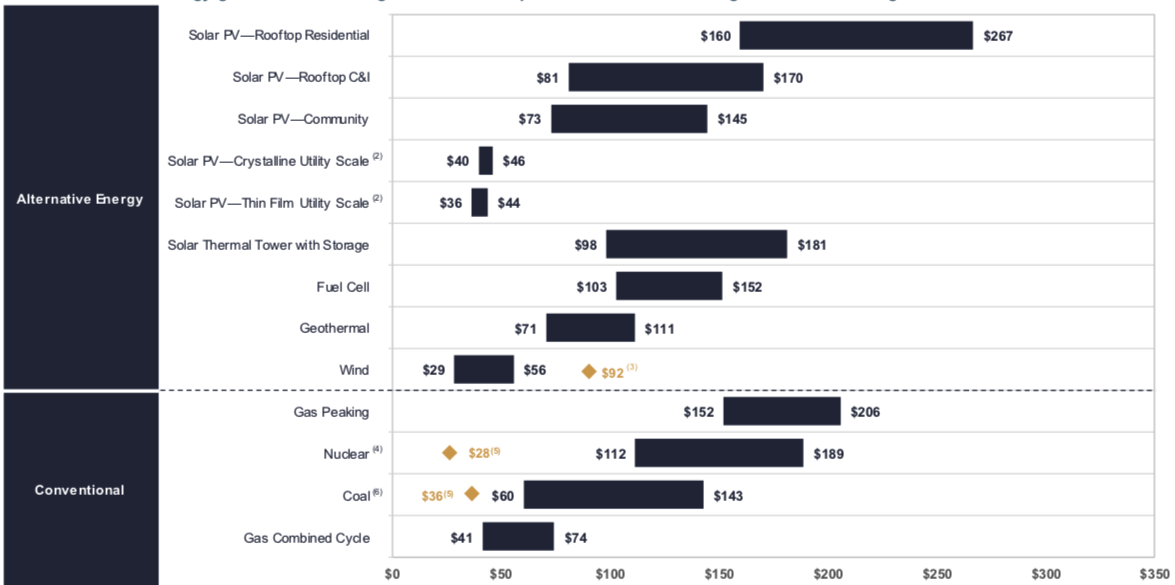
Source: Lazard
Note: Click on Lazard.com (page 2) for all footnotes.
As you can see above, wind does particularly well, but certain forms of solar also do quite well as far as LCoE is concerned. Fossil fuels, on the other hand, do not compare very favourably in general.
The carbon footprint
Next, the carbon footprint. Considering how much of total anthropogenic CO2 emissions originate from burning fossil fuels, let’s establish the carbon footprint of the various energy forms that may replace fossil fuels, as that is likely to have a major say in terms of which direction we will be heading in the years to come.
When talking about the carbon footprint, researchers differentiate between the direct and the indirect footprint. The direct footprint includes only emissions coming from producing the electricity. The indirect footprint also includes the energy needed to build the power stations and the energy required to run them. Researchers call that the embodied energy use.
The research I have come across show that the direct carbon footprint from nuclear, wind and solar is much lower than it is from other primary energy forms (Exhibit 7). Having said that, supporters of fossil fuels often argue that, once you include the indirect footprint, fossil fuel-based electricity is far more attractive.

Notes: BECCS = Bio-Energy with Carbon Capture and Storage, CSP = Concentrated Solar Power energy, PV = Solar Photovoltaic energy
Source: CarbonBrief.org
A study from CarbonBrief.org from December 2017 proved this to be pure fantasy, though. As you can see in Exhibit 8 below, the study found that, in conventional powerplants (coal, gas, bioenergy or hydropower), 10-20% of the lifetime electricity production is offset by the energy needed to build those power station and to supply the fuel. Those numbers are reduced dramatically when the electricity is produced by nuclear, wind or solar.
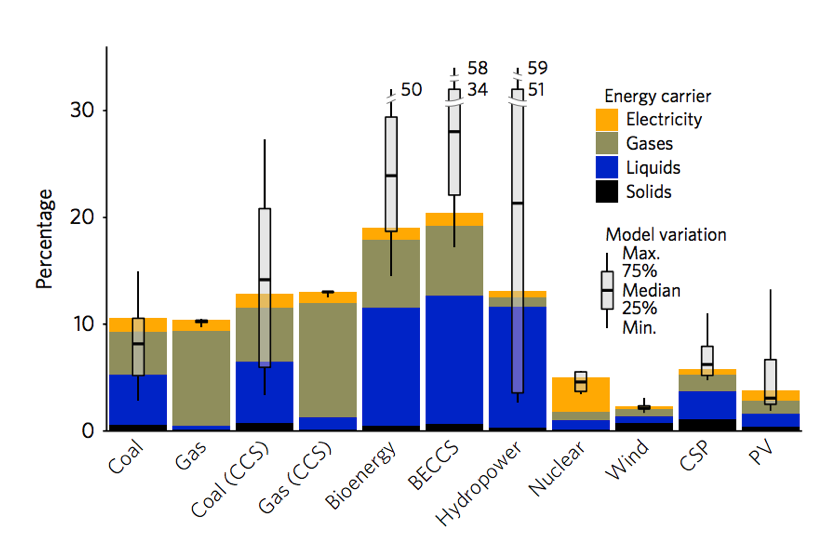
Notes: BECCS = Bio-Energy with Carbon Capture and Storage, CSP = Concentrated Solar Power energy, PV = Solar Photovoltaic energy
Source: CarbonBrief.org
The conclusion is therefore pretty obvious. From a carbon footprint point-of-view, wind is the most attractive primary energy form, but solar and nuclear are both vastly more environmentally friendly than any other energy form that we know of today.
Safety issues
Contrary to public belief, nuclear power is far safer than fossil fuels (Exhibit 9). That said, it is perceptions that dictate policy – not reality. Despite reliability being at an all-time high, most people still associate nuclear with the occasional disaster. A meltdown of reactor number 4 at Chernobyl in 1986 caused the biggest nuclear-related incident the world has experienced since World War II, and people still remember the events of 1986 vividly.
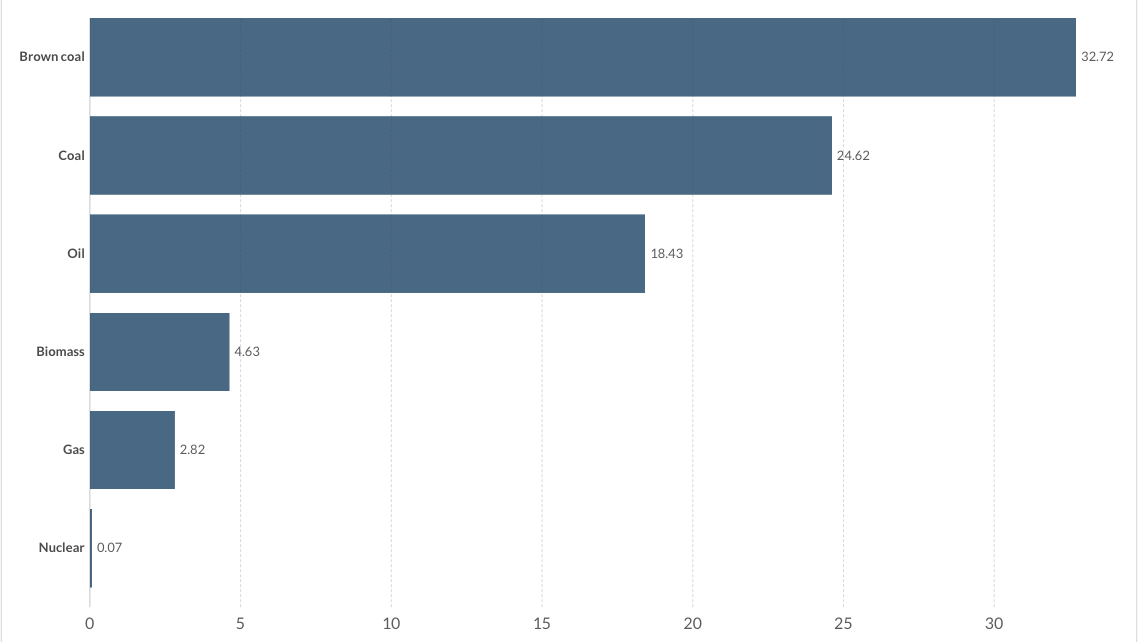
Source: Our World In Data
One more point to make re Exhibit 9 – when I decided to include this chart, some of my colleagues thought fossil fuels look so bad only because it is a massive ‘silent’ killer, i.e. people die from poor air quality, etc., but that is not the case. The deaths included in Exhibit 9 include only deaths in connection with the mining, exploration or transportation process.
As far as Chernobyl is concerned, estimates on how many actually died vary greatly, but everybody agrees that the number of deaths in and around Chernobyl were in the thousands (Exhibit 10). Now, some 34 years later, there is still an exclusion zone in place around Chernobyl and, according to Ihor Gramotkin, Director of Chernobyl, it will take at least 20,000 years before the Chernobyl site becomes habitable again.
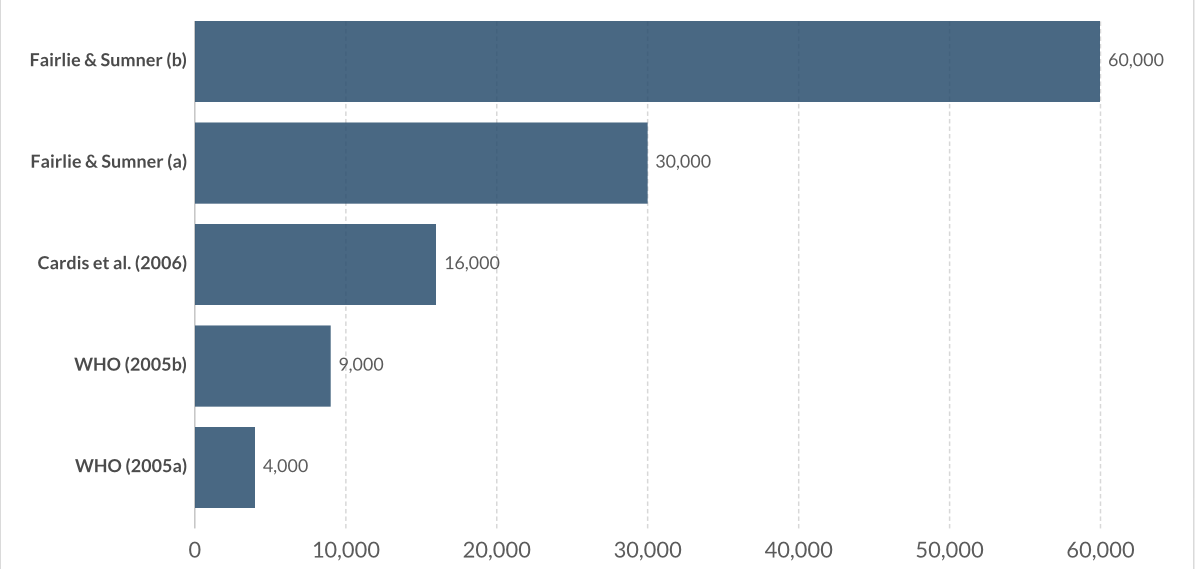
Source: Our World In Data
Despite the extent of the Chernobyl accident, the nuclear industry continued to grow, but that all came to an abrupt halt with the disaster in Fukushima in 2011. Following a major earthquake off the coastline of Japan, a 15-metre tsunami disabled the power supply and the cooling of the three reactors, and a partial meltdown followed. Following this latest accident, many countries in the old world turned against nuclear – most notably Germany which has now closed down all its nuclear reactors.
The simple choice and why it is not so simple
Adding those three issues up – cost, carbon footprint and safety – the obvious choice seems to be more wind – comparatively low LCoE, minimal carbon footprint and few safety issues, but there is more to the story than I have shared with you so far.
Back to the earlier question – why is there such a powerful link between electricity prices and the penetration of wind and solar? The best answer I have come across so far comes from the German researcher, Lion Hirth, who spelled it out 6-7 years ago. According to Hirth, the economic value of both wind and solar drops dramatically as their share of the power mix increases, and the reason is simple.
As Hirth said in his 2013 paper, both wind and solar are unreliable fuels with low capacity factors. (The capacity factor is the ratio of actual electricity output as a % of peak output.) Take for example solar. The sun is down many hours every day, and low on the horizon or obscured by clouds for a large fraction of daylight hours, which is why a capacity factor in the range of 10-25% for solar is perfectly normal. Importantly, the capacity factor does not improve as the technology is enhanced. It is an entirely exogenous variable.
The capacity factor for wind is, on average, a little higher than it is for solar but is still quite low, which is the key reason economic factors favour wind over solar in most countries. According to MacroStrategy Partnership, in North America, the capacity factor for wind is about 33% vs. 21% for solar. The corresponding numbers in Europe are 24% and 12% respectively.
A practical implication of the low reliability of renewables is that, sometimes, you generate too much electricity and sometimes not enough. In Denmark, where about 55% of the primary energy now comes from renewables (mostly wind), this is already a problem. Danish power stations have had to give electricity away for free to neighbouring countries when demand hasn’t met output. That has typically been the case during evening and night hours where demand is comparatively low.
Another implication is that, as the penetration of renewables increases, more backup shall be required. Why? Because you cannot run the electricity infrastructure on averages but need to cover the entire range of possibilities, which is why the actual price of electricity based on renewables is much, much higher than what first meets the eye. According to Andy Lees of MacroStrategy Partnership (I haven’t checked his calculations), electricity prices based on renewables are in fact more than five times higher than those based on fossil fuels. Therefore, using renewables in the process of generating energy is a massive misallocation of capital and may be one of the key reasons why Europe continues to underperform the US as far as GDP growth is concerned.
Going back to Lion Hirth, in his 2013 paper, he predicted that the economic value of wind on the European grid would decline as much as 40% once it makes up 30% of the energy mix in power plants, while the value of solar would drop by no less than 50% when it got to just 15% (source: Forbes – see Exhibit 11). Hirth predicted that, as our reliability on renewables increases, electricity prices will rise dramatically which will hold back economic growth for many years to come. Time has proven him right.
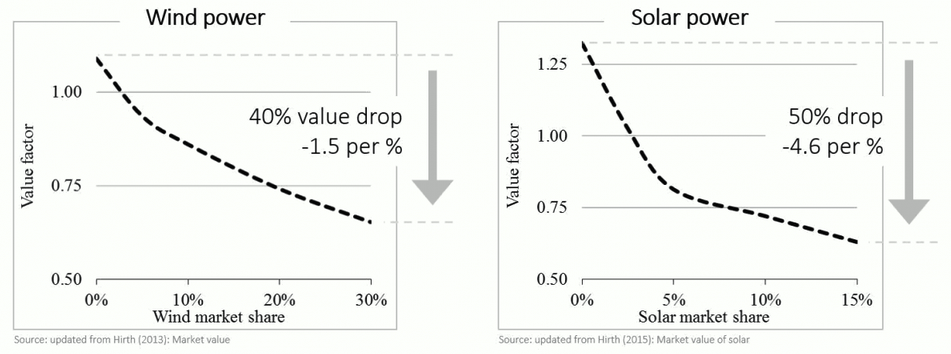
Source: Forbes
I should also point out that the analysis behind the LCoE calculations in Exhibit 6 does not include those hidden costs, making renewable energy forms appear more attractive from an economic point of view than they actually are. It is therefore no coincidence that the two countries in Europe with the highest share of renewables in the power mix, Denmark and Germany, also have the highest electricity prices, and that is even after adjusting for taxes which are high – particularly in Denmark.
Also, and this may come as a surprise to some of our readers, there are in fact serious environmental and human costs associated with both wind and solar. Wind first. Wind turbines are known to cause significant psychological problems to neighbours – wind parks are actually surprisingly noisy – and many people who live close to one of those suffer from sleep deprivation and other issues. Problems like that have driven more and more new windfarms offshore. That may be a step in the right direction from a human/environmental point-of-view but, cost-wise, it is not. The installation cost of offshore capacity is more than twice onshore costs, and operating expenses in offshore wind parks are also dramatically higher (see here for details).
As far as solar is concerned, the story is even worse. Let me share with you what Andy Lees of MacroStrategy Partnership said to me when I prepared for this paper:
It may be a solution to atmospheric carbon problems, but for nature and the ecosystem as a whole, it is almost certainly far more destructive than fossil fuels. Obviously land supports plants and animals etc., but a large part of that is the land being a receptor for the sun’s energy which, if you take it away, you are taking it away from plants and animals etc., so it’s no different to taking massive areas of land from nature. Also, by covering the ground with solar cells, you turn the ground beneath into desert and thereby stop it from absorbing water, thereby exacerbating your water shortage.
As you may recall from Exhibit 1, renewables (ex. hydro) account for less than 10% of our primary energy today, and I would be hugely surprised if we ever reach anything even remotely close to 100%. The current move towards renewables is obviously politically driven but, sooner or later, the penny will drop. For the reason stated by Lion Hirth, you don’t want renewables’ share of the power mix to get too high – at least until we get better at storing electricity. Therefore, if fossil fuels increasingly are an F-word, something else shall be required. More on that below and in part II of this paper.
The solution?
If renewable energy is not the magic solution it is often portrayed as, and if fossil fuels will most likely be phased out in the years to come, it is only fair to ask what should then make up the difference? (In part II of this paper, I will go into more detail on current research into power storage and new fossil-free energy forms.)
Given the vastly improved safety record, one could argue that traditional nuclear could bridge that gap, but safety – at least perception-wise – seems to be an insurmountable problem. Until such time that a solution can be found, fossil fuels are therefore likely to be the default choice in many countries, and that is despite oil, gas and coal doing immense damage to our environment.
Another issue to bear in mind is that traditional nuclear can never be the marginal provider of electricity as you cannot temporarily reduce power generation in a nuclear reactor. It is all or none, as re-starting a nuclear reactor after a shutdown costs hundreds of millions of dollars. Shutting it down temporarily is simply not an option – it is far too expensive.
This would pose a problem for countries that get most of their electricity from a combination of renewables and nuclear. What if demand suddenly drops sharply under normal wind conditions, as it did in the early days of the COVID-19 crisis? A combination of nuclear and renewables is not a good combination, unless you generate a reasonable amount of energy from another energy form which can then act as the swing factor.
The long-term fix to this problem is the eventual rollout of fusion energy which will be discussed in more detail in part II, but fusion energy cannot be relied upon any time soon, as it is still at least 10-15 years away. Something else is needed, but what?
Thankfully, the industry is onto something that could potentially change the power mix dramatically – something called SMRs or Small Modular Reactors. An SMR is a small nuclear power reactor that stands out from conventional nuclear power reactors in several ways. As an SMR reactor requires much less space, it can slot into a brownfield site in place of a decommissioned coal-fired power plant. Most importantly, an SMR nuclear power station requires no safety zone as it cannot melt down, assuming the architects behind the SMR concept are to be believed. Nuclear power stations could therefore be moving into the suburbs a few years from now.
The architecture behind the reactor modules is such that, instead of building the SMR reactor on-site, it can be built by advanced robotics in a factory, reducing human error to 0%. Furthermore, the technology is such that the reactor can be located either underground or underwater if warranted. Given those added safety features, even countries which have refused to go for conventional nuclear, could possibly embrace this technology.
An SMR is typically capable of generating up to 400 Mwe but can do much less if needed. The standard set-up is 12 modules next to each other, but that is easily reduced. As the average coal-fired power plant that is currently being decommissioned generates 145 Mwe, it would be relatively straightforward to swap from coal to SMR nuclear.
A US company called Nuscale Inc. is currently working with the US Nuclear Regulatory Commission to land its first SMR certificate. Phase four of the review and approval process has just been completed, and the company expects to receive the regulatory go-ahead in late 2020 for the first commercial SMR to open in Idaho in 2027.
There are several caveats, though. Firstly, nuclear is still perceived to be dangerous, SMR or not. It will take a massive information campaign – and probably quite some time – to turn a very nuclear-sceptic public around.
Secondly, it is still early days and, as stated above, the final permission to go ahead in the US has not yet been granted. That said, many other countries have their own SMR programme. China, Canada, Russia, the UK (through Rolls-Royce), France, India and the Czech Republic are all at various stages of developing alternative SMR platforms.
Thirdly, cost remains a big issue. For SMRs ever to become economically competitive, they need to be built in large numbers, and all the countries mentioned above need to settle on a common design which would reduce costs dramatically. In terms of scale, there is plenty of appetite for nuclear outside Europe and the US – particularly in Asia but also in other parts of the world.
There is another way to make SMRs profitable, though, which has to do with the adaptability of the SMR design. It would be possible not only to use an SMR reactor to generate electricity for the grid, but also to produce hydrogen for fuel or to desalinate water. According to Nuscale, using excess energy for desalination may dramatically improve the economics of an SMR. More on hydrogen in part II.
Finally, and that may be the biggest problem of them all, the SMR technology may have addressed important safety issues, but the nuclear waste problem remains. According to proponents of the SMR technology, waste is less of a problem, but sceptics say there is no proof of that.
Summing it all up
Given all of the above, what is the primary energy mix likely to look like ten years from now? The easy one first. If you take another look at Exhibit 1, you can see that coal accounts for over one-quarter of the mix today, and coal will likely be the first fossil fuel to be phased out. I doubt it will all have happened by 2030, but coal will almost certainly account for much less of the power mix in 2030 than it does today. Gas, although less damaging to our environment than coal, will go the same way over time. Between them, coal and gas account for half our primary energy consumption.
Oil is a slightly more complex story, as significant amounts of oil is used for other purposes every day, e.g. to produce various plastic products. Consequently, the impact on oil prices will be less dramatic than on coal and gas prices, but the impact can only be expected to be negative.
Many seem to believe that renewables will eventually make up most of our energy mix but, for all the reasons stated above, I don’t think that is likely to happen anytime soon. I will have to qualify that statement, though. The day technological advances allow us to store electricity more effectively, the use of renewables will likely increase further, but there are no signs of that happening anytime soon, hence the need for something else.
Assuming fossil fuels will indeed be phased out, and assuming there isn’t suddenly a miraculous breakthrough in the development of fusion energy, there is therefore only one feasible alternative to fossil fuels, and that is fission energy, or nuclear as we know it today. I very much doubt that the public anywhere in the old world will suddenly warm to conventional nuclear, which is why the SMR project is so important, not only for the nuclear industry but also for the wellbeing of planet Earth. Huge resources will therefore be spent on overcoming the remaining hurdles.
In part V (to be published later this year), I will dig into more detail on the investment implications of all of this. For now, I will simply summarise my finding so far.
Fossil fuels will increasingly be phased out of the primary energy mix, but it will happen at a very different pace from country to country. Renewables seem to be the obvious choice from a political point-of-view but is far from the optimal solution economically. One could even argue that, environmentally, there are some serious questions to be addressed when adopting wind and solar at a massive scale.
Traditional nuclear is not an option. It would be akin to political suicide in many countries to scale up that programme. That raises another issue, though, and one which I haven’t touched on yet. Many nuclear power stations around the world are fast approaching the end of their life and will have to be decommissioned in the not so distant future. What will happen then? I suppose – but it is only a guess – that SMR nuclear will replace most of the old-fashioned nuclear reactors which will close down in the years to come.
Consequently, on the negative side, fossil fuels stand out. They will most likely be the big loser longer term. On the positive side, for a mix of economic and political (mostly environmental) reasons, SMR nuclear and wind stand to win with solar coming a distant third. And, given the negative impact the use of renewables have on productivity growth (much misallocated capital), the bigger the share of the power mix that comes from renewables, the more productivity growth will struggle.
Niels C. Jensen
23 June 2020
PS. Thank you to Andy Lees from MacroStrategy Partnership for assisting me on some of the more technical aspects of this paper.