Thorium
Issues to be addressed in this research paper
There are two reasons why I am writing a paper on thorium now. First and foremost, I have been bullish on uranium for a while and argued that, over the next 5-7 years, there is little on the horizon which will prevent uranium prices from going higher. Secondly, an existing ARP+ subscriber contacted me recently and asked for my views on thorium. Essentially, he had the same concerns – will thorium eventually replace uranium as fuel in nuclear reactors? In this paper, I will compare the two technologies and try to establish which one is likely to prevail.
Uranium (spot) was trading around $24/lb when I first zoomed in on the opportunity (see the research paper here) and is now slightly above $32/lb. However, given the large number of nuclear power plants coming onstream over the next few years combined with flat uranium supplies (more on that later), uranium prices should go up a fair bit more.
Thorium has entered the picture now, and at least some investors are of the opinion that it may increasingly replace uranium – the fuel in almost all nuclear reactors today. Although the advantages of thorium are indeed many, there are also issues that one shouldn’t ignore – issues that I will discuss below.
What is thorium?
(The following few paragraphs are essentially a copy and paste from World Nuclear Association’s 2020 update on thorium.)
Thorium (Th-232) is a metal which was discovered in 1828 by the Swedish chemist Jöns Jacob Berzelius. He named it after the Norse god of thunder, Thor, hence the name. It is found in small amounts in most rocks and soils, where it is about three times more abundant than uranium. Soil contains an average of around 6 parts per million (ppm) of thorium. It is very insoluble, which is why it is plentiful in sandy soils but not in seawater, in contrast to uranium.
Thorium is only modestly radioactive and is not fissile – i.e. not able to undergo nuclear fission – on its own. Therefore, it can only be used in nuclear reactors in conjunction with a fissile material. The only fissile materials used today are U-233, U-235 and Pu-239. Having said that, it is fertile and, upon absorbing a neutron, will transmute to U-233, which is an excellent fissile fuel material. To use thorium as fuel in nuclear reactors therefore requires that Th-232 is first irradiated.
When pure, thorium is a silvery white metal that retains its lustre for several months. However, when it is contaminated, it slowly tarnishes, becoming grey and eventually black. When heated, it ignites and burns brilliantly with a white light. Thorium oxide (ThO2) has one of the highest melting points of all oxides (3,300°C) and has therefore found plenty of applications, for example in light bulbs, heat-resistant ceramics and in various scientific instruments.
To continue reading...
The most common source of thorium is a rare earth phosphate called monazite which contains, on average, 6-7% thorium. Monazite is found in rocks, but the richest concentrations are in sandy soils. At present, monazite is extracted in only a small handful of countries – India, Brazil, Vietnam and Malaysia – and in very modest amounts, less than 10,000 tonnes annually in total.
Without commercial rare earth recovery, thorium production does not make any economic sense. According to the World Nuclear Association, extraction of thorium as a by-product of rare earth elements from monazite is probably the way forward, if the objective is to make thorium extraction economically viable.
To recover thorium from monazite usually involves leaching with sodium hydroxide at 140°C, followed by a complex process to precipitate pure ThO2 which is relatively inert and does not oxidise further, unlike uranium dioxide (UO2). It has higher thermal conductivity and lower thermal expansion than UO2, as well as a much higher melting point, all of which can be taken advantage of.
World thorium reserves are estimated to be approx. 6.5 million tonnes, 13% of which can be found in sand deposits on the south and east coasts of India. India is far from the only country having rich thorium reserves, though, see Exhibit 1 below.

Source: Word Nuclear Association
Why thorium?
As a nuclear fuel, thorium is thought to have several advantages over uranium. It is more abundant, much harder to weaponise, and is less prone to meltdowns as it operates under much lower pressure than uranium. The main advantage of thorium, though, is that most nuclear waste from thorium-fuelled reactors has a half-life measured in dozens of years rather than thousands of years as in the case of uranium. Let me explain.
At the heart of the design is a molten salt fast reactor, where the core of the reactor is filled with Th-232, U-233 and various fluoride salts. As fission occurs, heat is released from the core of the reactor and absorbed by the surrounding salt. The salt melts into a molten state, which runs a heat exchanger (at about 750˚C), which again drives a turbine to generate electricity. The radiated salt flows into a post-processing plant, which separates the uranium from the salt. The uranium is then sent back to the core to start the fission process again (source: Powergen International).
These types of reactors are also called LFTR reactors (Liquid Fluoride Thorium Reactors). It is a modified version of a reactor that operated in the Oak Ridge Lab in the USA in the 1960s. In the new (French) version, the core of the reactor is a two-metre high and wide steel cylinder filled with molten salt, Th-232 and U-233 as fissile fuel.
There are five, uranium-fuelled reactor types already in operation which, if slightly modified, could use this technology (source: Forbes). A thorium–fuelled reactor operates at much lower pressure than uranium-fuelled reactors do, virtually eliminating the risk of meltdowns. As I said earlier, thorium itself is not fissile but, when bombarded with neutrons, it transmutes to U-233, which is. Fission products (hazardous waste) are continuously removed from the molten salt reactor. After ten years, most of these are hardly radioactive any longer. Having said that, about 20% of the nuclear waste must be stored for 300 years and a few percent for much longer.
Furthermore, thorium-fuelled reactors do not require massive cooling, meaning they can be air-cooled and placed anywhere. Also, it is claimed (but has not yet been proven) that a single tonne of thorium burned in a molten salt reactor can produce one gigawatt of electricity. By comparison, a conventional uranium reactor would need to burn 250 tonnes of uranium to produce the same amount of energy. It is expected that a thorium-fuelled reactor will operate at about 45% thermal efficiency, meaning it is about 20% more efficient than a conventional, uranium-fuelled nuclear reactor.
Cost-wise, if thorium-fuelled reactors become prevalent, the cost – which is considered prohibitively high at present – will decline, as thorium is widely available anywhere in the earth’s crust. In the past, thorium has been tossed aside as a by-product of rare-earth metal mining. If the concept is rolled out more widely, there is enough thorium around to generate the energy mankind needs for thousands of years.
…and why not
Thorium cannot in itself power a reactor. Unlike uranium, it does not contain enough fissile material to initiate a nuclear chain reaction. As a result, it must first be bombarded with neutrons to produce the radioactive isotope U-233. In other words, as critics argue, a thorium-fuelled reactor is in fact a U-233 reactor. This isotope is more hazardous than the U-235 isotope used in conventional, nuclear reactors, as it produces both U-232, technetium-99 and iodine-129. The half-life of U-232 is no less than 160,000 years, the half-life of technetium-99 up to 300,000 years and the half-life of iodine-129 a whopping 15.7 million years, i.e. a thorium-fuelled reactor still produces highly radioactive waste, although in smaller amounts.
Finding a way to produce the fuel economically is another big challenge, mostly because thorium’s melting point is so high – the highest of all oxides. Even if the technology progresses to the point where it is commercially viable, it will face the same problems as conventional nuclear – it is not renewable, nor is it sustainable, and the technology is not tried and tested, which conventional nuclear is. Moreover, the main players are not interested in the technology, critics say.
Neither are politicians, one could argue. A handful of US attempts to fund research on thorium-fuelled reactors have fallen flat, but a bill passed by the US Senate in July 2020 – the so-called Nuclear Energy Leadership Act – called for the development of two advanced nuclear reactors by 2025 and another five by 2035. Thorium is not specifically mentioned in the bill, but a thorium-fuelled reactor could quite possibly be one of the reactor designs which will be explored.
Why there is no alternative to nuclear
Nearly every country on planet Earth – 197 in total – have signed up to the so-called Paris Agreement – a UN-sponsored political agreement entered into in Paris in December 2015 which aimed to limit the rise in the global average temperature to 2˚C above pre-industrial levels. Subsequently, the limit has been reduced to +1.5˚C.
Although I shall not, at least not in this paper, discuss the Paris Agreement in any detail, the harsh reality is that unless fossil fuels are completely phased out relatively soon, neither +1.5˚C nor +2˚C are realistic targets. Many scientists even argue that it is already too late. Even if fossil fuels were abandoned tomorrow morning, we have already passed the point of +2˚C, they say.
The sun doesn’t always shine and the wind doesn’t always blow, i.e. renewable energy sources are not reliable. (The technical term is “too intermittent”, meaning they are not reliable, but it sounds better.) Therefore, unless (until) the energy storage technology improves, a modern economy cannot afford for renewables to account for too much of the primary energy mix. That would make the economy far too dependent on weather patterns. In other words, given the technology in place today, if fossil fuels were to be phased out, whether environmentalists like it or not, the only realistic alternative is therefore nuclear.
(I am often accused of being against renewables by saying this, but I am not. I am just stating a simple fact.)
Commercial thorium projects currently underway
Thor Energy of Norway (see here) was the first company worldwide to produce energy through thorium but, for reasons unknown to me, the company has not made significant progress in recent years and faces rising competition from other parts of the world.
India is at the very front of that curve. For decades, India have allocated substantial resources to research into thorium-based nuclear energy, reason being that the country is so rich on thorium reserves. Indian nuclear scientists have already designed a reactor that is designed to run on thorium. The country has several test (research) reactors already up and running and plan to launch the first commercial thorium-fuelled reactor before the end of this decade.
China is another major player in the thorium space, having committed $3.3Bn to two molten salt thorium nuclear reactors in the Gobi Desert (see here). The Chinese hope to have the two reactors delivering electricity to the grid in the next few years. In Indonesia, ThorCon and the country’s government are working together to launch a thorium molten salt reactor, expected to be operating by 2025 (see here).
Thorium Power Canada, in partnership with DBI (see here), develop thorium reactor designs, including a planned 10 megawatt reactor in Chile. According to Thorium Power Canada, that reactor will provide enough power to produce 20 million litres of freshwater per day at a Chilean desalination plant. The electricity output from that reactor is equivalent to powering 3,500 private homes.
Most other thorium projects around the world are still at the research stage, and only limited amounts of private capital have been invested. In most countries, research into thorium-fuelled reactors is almost exclusively funded by the public sector, limiting the number of investment opportunities.
Where do we go from here?
As I have said before, the long-term solution to the world’s energy needs is an emerging technology called fusion energy – a nuclear technology that works fundamentally differently from the nuclear technology we adopt today, called fission energy. That said, fusion energy is at least 10-15 years away, and we cannot afford to wait that long to phase out fossil fuels if we want to get the mounting climate crisis under control. Having said that, if fusion energy is not an option in the short to medium term, what is? As far as I can judge, only two options are available – uranium or thorium-fuelled fission reactors.
A growing number of governments around the world realise that. According to World Nuclear Association, 42 nuclear reactors will come into operation between now and 2025, mostly in Asia (Exhibit 2). Another c. 100 nuclear reactors are on order, and a further 300+ have been proposed. In addition to those programmes, 30 countries around the world are considering going nuclear. You can see the list here.
Every single, commercial power plant in operation today and every single new reactor coming onstream over the next 4-5 years (see Exhibit 2 again) is uranium-fuelled. Only a handful of research reactors currently in operation are thorium-fuelled. As already pointed out, India is the country most committed to the development of thorium-fuelled reactors, which is a natural consequence of the vast thorium reserves the country possesses. The plan is to go live with its first commercial, thorium-fuelled reactor before the end of the 2020s. That said, India already has 20 uranium-fuelled reactors up and running, another six under construction, 17 planned, and 40 proposed. In other words, India is committed to nuclear – not just to thorium.

Source: World Nuclear Association
In the case of China, just like in India, for every thorium-fuelled reactor coming onstream over the next few years, there are many uranium-fuelled reactors waiting to go live. China has only 15 nuclear reactors in operation today but has 26 under construction, 51 planned, and 120 proposed – all but the two in the Gobi Desert being uranium-fuelled.
Will uranium or thorium prevail?
In order to understand the impact a gradual rollout of the thorium technology may have on uranium prices, it is important to understand both the demand side and the supply side. Let’s begin with demand. Demand for uranium is highly predicable, and there are two reasons for that. Firstly, uranium-fuelled power plants never shut down, as it is far too expensive to start them up again, meaning that you know almost to the second decimal point how much uranium existing nuclear power plant shall require in the foreseeable future. Secondly given the long lead time from planning to going live, we also know how much demand for uranium will increase over the next decade or so.
Most existing uranium mining companies are unprofitable at current prices, and they are adamant that mining will not be increased unless uranium prices improve dramatically. Should uranium prices rally sharply, as they last did in 2006-08 (with only modest impact on electricity prices as the cost of fuel accounts for less than 10% of total costs in nuclear power plants), new mining capacity will undoubtedly be added, which will most likely bring an end to the good times. The average incentive price to establish additional uranium mining capacity is about $60/lb, though, so still almost double current levels.
As you can see in Exhibit 3 below, by 2023, demand will begin to outstrip supply. As thorium-fuelled reactors on a meaningful scale are at least 8-10 years away and probably even longer, given the mixed reception, uranium will not face any meaningful competition from thorium until 2030 at the earliest. Furthermore, given that fusion energy is probably no more than 10-15 years away, and that all fission technologies, whether uranium or thorium-based, will be hopelessly outdated once fusion is here, I am not even sure thorium will ever be rolled out in size.
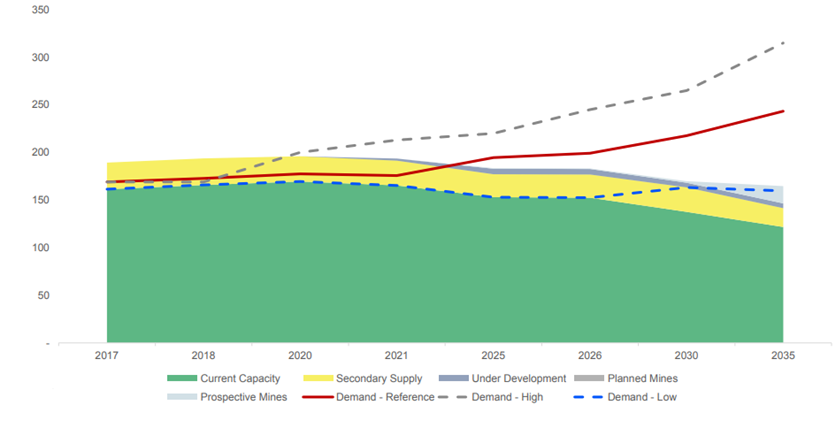
Source: Yellow Cake PLC
One could argue that, given how difficult – almost impossible – it is to turn the fissile materials from a thorium reactor into nuclear weapons, the world would probably sleep better if totalitarian regimes like North Korea were allowed to install thorium reactors but not uranium reactors. On the other hand, one could also argue that many countries will never replace their existing network of uranium-fuelled reactors, as they need the uranium for their nuclear weapons programme.
As Forbes argue:
Had it not been for mankind's seemingly insatiable desire to fight, thorium would have been the world's nuclear fuel of choice. Unfortunately, the Cold War pushed nuclear research toward uranium, and the momentum gained in those years has kept uranium far ahead of its lighter, more controllable, more abundant brother to date.
Investment considerations
Let’s begin with the easy one – how do you invest in thorium? You don’t! I am not necessarily suggesting it will be a bad investment – not at all, but most R&D into thorium is conducted by government-controlled research groups, so there is little you can invest in (yet). To the best of my knowledge, there is only one publicly traded company working on the thorium technology, and that is an American company called Lightbridge Corporation which is listed on Nasdaq with the ticker symbol LTBR. Lightbridge has the advantage of being an early mover, but the fact that there is a scarcity of competitors is a strong hint that it is still too early to invest.
As far as uranium is concerned, we know that, despite all the negative sentiment in Europe and North America, demand for uranium will rise meaningfully over the next 5-10 years. We also know that uranium mining companies are reluctant to add to their mining capacity unless uranium prices rally to about twice current levels, which is the price the industry typically needs to make a profit. In other words, the demand/supply equation will most likely shift over the next few years in favour of higher uranium prices. Furthermore, we also know that demand for uranium is almost entirely inelastic, as you never shut down a uranium-fuelled reactor – not even in deep recessions. It is far too expensive to start it up again.
Knowing all of that, it is a relatively safe bet when I predict uranium prices to go higher in the years to come. You can follow the uranium market here. By far the simplest way to invest in uranium is through a UK listed company called Yellow Cake PLC (see here). Yellow Cake is the broker between, on one side, the uranium mining industry and, on the other side, licensed buyers of uranium, be it nuclear power plants or nuclear research centres. Yellow Cake’s stock price is driven by the intrinsic value of its uranium holdings, i.e. the two most important drivers of the stock price is the uranium spot price and the USD/GBP exchange rate. The stock currently trades at a very modest premium to its net asset value (c. 3%) and, by buying Yellow Cake rather than a uranium mining company, you have none of the execution risk that typically follows mining companies.
Sceptics often argue that unconventional uranium, particularly uranium recovered from phosphate rock when producing phosphoric acid, is plentiful and could overwhelm the market. Should that happen, the supply/demand balance would be badly affected, critics say. While correct that phosphorite deposits contain significant uranium resources (millions of tons), it is at a very low grade (0.005-0.015%), and the research team at Bank of American Merrill Lynch estimate that uranium from phosphate rock would increase global uranium supplies by 2% at most.
Final few words
Based on my findings, I conclude that investing in thorium is premature, that investing in uranium still makes sense for at least another 5-7 years, even if certain countries begin to switch to thorium-fuelled reactors, and that renewable energy forms will find their natural place next to nuclear energy in a fossil fuel-free world, but that you shouldn’t expect renewables to account for anything close to 100% of our energy mix until the energy storage technology improves.
One such improvement could be the ability to convert electricity from wind or solar to liquid hydrogen, which would allow trucks, trains and aircraft to be fuelled 100% by green energy. I am sure we’ll get there one day, but we are still years away. Only a couple of months ago, I discussed this topic in a research paper on hydrogen – see here.
Niels C. Jensen
19 July 2021